About Our Projects
We aim to better understand how bacterial and mammalian bioprocesses are coordinated in the living cell, and how pathways are regulated on the physical (protein interactions) and functional (gene dependencies) levels. This requires exhaustive analysis of a collection of high-throughput omics data, such as proteomics, network biology (i.e., interaction networks), quantitative genetics, comparative genomics, transcriptomics and overlaying these multiple datasets often reveals key molecular attributes linked to disease phenotypes, which essentially expedites biomarker discovery coupled with the development of innovative therapeutic interventions.
Proteomics and Network Biology
We continuously uncover protein signatures associated with neurological disorders or infectious diseases in different cell or tissue types. As well, we extensively map protein interactions in the bacterial cells and mitochondria extracted from stem cell-differentiated neuronal cell types associated with select human diseases. This is achieved upon large-scale isolation and subsequent mapping of endogenous soluble or membrane protein complex compositions at near physiological conditions. We routinely implement cutting-edge approaches that combine HPLC-operated biochemical fractionation or affinity purification with precision mass spectrometry.
Currently, Babu’s laboratory leverages these approaches in neuron-like cell lines or brain organoids to understand critical mitochondrial mechanisms in health and disease, and how mitochondrial dynamics and energetics are impaired in complex neuropsychiatric, neurodegenerative, and rare metabolic disorders. Mapping the underlying molecular mechanisms in patient samples vs. healthy counterparts’ sheds light on how proteomic landscapes and interaction networks change in human disease, thereby accelerating areas such as drug discovery and precision medicine.
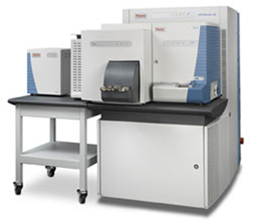
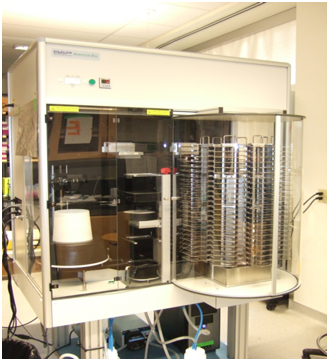
Quantitative Genetics
The effective contribution of “omics” to biomedical research can be garnered by combining multiple approaches and although physical networks can highlight key protein interactions that drive human disease, they do not necessarily reveal pathway relationships. Genetic screening in contrast, where the growth defect of a double mutant is compared to the fitness of the corresponding single mutant counterpart, can elucidate functional relationships between genes.
Inspired by successes in other model organisms, we have systematically developed a high-throughput genetic screening approach for prokaryotes, termed E. coli Synthetic Genetic Array (eSGA). The powerful eSGA platform generates high-density functional association maps of genes involved in cell envelope maintenance or assembly, transcriptional regulation, and other pivotal bioprocesses in the cell.
We are also currently focusing on generating large-scale genetic interaction maps for essential biological processes and combining genetics and drug screening data to elucidate novel drug targets. We are also actively engaged in developing advanced analogous genetic interaction technologies for other prokaryotes and eukaryotes, including CRISPR-based methodologies for pathway analyses in human neuronal models of variants associated with neuropsychiatric and neurodegenerative disorders.
Comparative Genomics
Harnessing the complete power of omics additionally requires the integration of comparative genomics platforms of data for filtration purposes, and we utilize advanced computational frameworks and machine learning algorithms for processing, integrating, and interpreting large-scale interaction datasets to better understand cell physiology and minimize the central false positive data issues associated with omics approaches.
As well, we are investigating the evolutionary trajectory of biological pathways and complexes using comparative genomics to identify a spectrum of behaviors, including complexes that are highly modular for drug discovery.
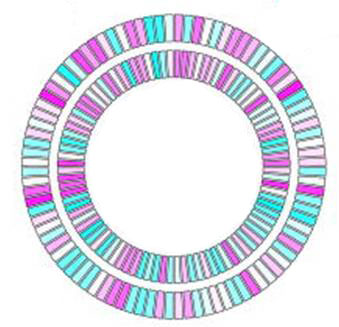